Extreme weather events coupled with increasing electric and thermal demands have exposed vulnerabilities in the existing infrastructure. The result has been major utility failures and interruptions that can have a detrimental impact on many facilities. The importance of evaluating and improving resilience and redundancy at your facility is critical. One solution to support resilience is on-site generation.
Defining Resilience vs. Reliability
Conversations around reliability and resilience are key drivers when identifying risks and methods for mitigation related to utility infrastructure interruptions. While resilience and reliability are related, it is important to understand the differences between the two:
Resilience: Based on the Presidential Policy Directive (PPD) 21, resilience is defined as “the ability to prepare for and adapt to changing conditions and withstand and recover rapidly from disruptions.” Resilience includes “the ability to withstand and recover from deliberate attacks, accidents, or naturally occurring threats or incidents.”
Reliability: As defined by the Department of Energy (DOE), reliability is “the ability of a system or its components to adapt to changing conditions and withstand and rapidly recover from disruptions.”
In other words, resilience is mitigating risks related to large-scale infrequent major events, whereas reliability mitigates risks associated with frequent, smaller events that impact facilities.
Creating Resilience Objectives
Much like sustainability was 20 years ago, resilience has become a lens through which we look for project success; it should be incorporated into every project. When considering resilience, key objectives include:
1. Developing a comprehensive approach to assessing risks that could influence your systems and operations.
2. Redefining risk to be forward-looking rather than backward-looking. Historical context and trends are useful, but utility enhancements need to accommodate the future.
3. Recognizing the lag of regulatory framework to consider that current regulatory standards are likely outdated. Often, projects are being developed today that respond to issues from 30 years ago.
In the context of facilities, resilience is best defined by what it provides—risk mitigation. Risks are different in every situation and can vary over time, so it’s critical to understand what unique risks or threats exist at your facility or campus and how they could impact the overall mission. Below are a few examples of possible threats related to an electric outage:
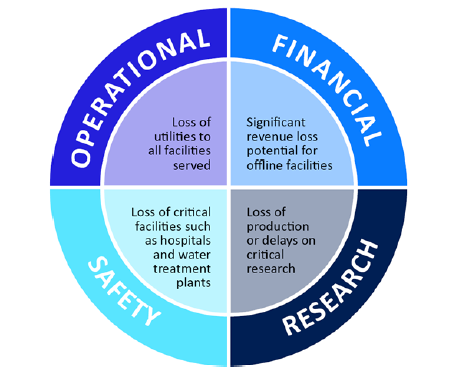
On-site Generation
Critical facilities such as hospitals, research facilities, and public buildings often have some form of emergency generators to provide backup power to critical assets in the event of an outage. When properly applied, these assets can provide additional benefits beyond backup power, including electric demand offsets, improved efficiency through heat recovery, and emission reductions. These can also be used to develop advanced microgrids capable of operating independently from the electric grid, which can bring further value by providing energy and cost savings as well as unmatched resilience. These benefits can improve the value proposition of a potential investment in on-site generation.
On-site generation technologies available on today’s market include:
Reciprocating Engines/Combustion Turbine Generators: These fossil-fueled assets are flexible resources that can respond to dynamic loads and are the anchor resource in modern electrical grids. They can be configured to produce power alone or configured as a combined heat and power (CHP) system whereby waste heat is captured to support the heating or cooling demands of your campus or facility, dramatically increasing the system’s economics.
Solar Photovoltaic/Energy Storage: Solar photovoltaic technology utilizes an array of solar panels to harvest the sun’s energy. Largely a decarbonization technology, solar is not well suited for backup power or microgrid application without some form of anchor resource but does work well in an advanced microgrid in concert with other resources. Pairing solar with energy storage technology can be a key component to improving the economics of system resilience.
Wind Generation/Energy Storage: Wind turbines convert wind energy to electricity. Like solar and energy storage, it can be a useful tool in supporting the economics and decarbonization of the grid, but it is not well suited as a resilient solution alone.
Fuel Cells: Fuel cells generate power through an electrochemical reaction, which mixes fuel with air, utilizing a catalyst to produce electricity. Fuel cells can operate on biogas, natural gas, or renewable natural gas. Fuel cells have high efficiencies ranging from 47-50% and emit low emission levels. They can be configured to produce solely power, but they also have the ability to capture additional heat from the process to provide steam or hot water in a CHP application.
Though several technologies exist, there is no one size fits all solution. Air permitting, load dynamics, response time, and electrical and thermal load phases are among the critical factors to consider when evaluating on-site generation. Your system should be evaluated by a professional with expertise in on-site generation technology to identify the right solution that aligns with the mission and goals of your facility.
Case Study: University of Minnesota
Jacobs worked with the University of Minnesota to create an on-site generation solution by developing a CHP plant that generates approximately 22 MW of electricity. The system employs a combustion turbine generator coupled with a heat recovery steam generator. To enhance resilience in the frigid Minneapolis winters, the combustion turbine is capable of operating on natural gas or fuel oil in case the natural gas supply is curtailed.
With critical facilities such as hospitals and research centers on campus, resilience is essential to the university. As such, reliability of the plant was vital, and considerations for redundant auxiliary equipment were incorporated into the design.
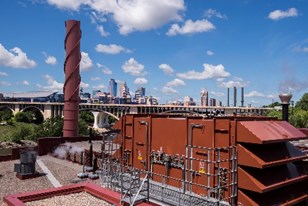
In addition to improving the resilience and reliability of their campus, Jacobs identified several financial and environmental incentives. By installing the new CHP facility, the university was able to offset purchased power and save approximately $5.4 million in annual utility costs. In addition, the project enabled a massive reduction in the campus carbon footprint, allowing for savings of more than 32,000 metric tons per year.
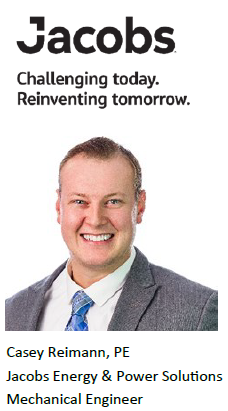